Dynamics of Dissipative Solitons in Femtosecond Enhancement Cavities
- Posted by
Red Pitaya Team , June 21, 2023
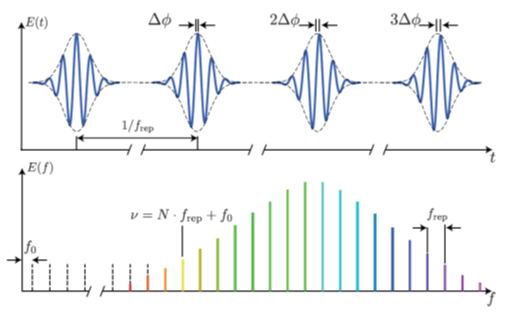
Even to experienced tech geeks, a physics master’s thesis can sound like Klingon, or worse. Already in the title of this text the term “solitons” isn’t one you come across daily, to say the least. Temporal cavity solitons (CS) are packets of temporally localized electromagnetic waves that maintain their constant shape (envelope) while they propagate through a nonlinear dispersive medium. In nonlinear optical cavities, they exist as temporally dissipative solitons, an asymptotically stable state of equilibrium of one or more pulses, which can circulate infinitely.
Temporal cavity solitons were first demonstrated in 2010 in continuous wave (CW) laser-driven passive fiber cavities. The great interest in solitons is due to their optical nonlinear properties and their use as passively mode-locked states for active laser cavities, making them a key component for ultra-fast optics and frequency-comb technology.
In his 2023 Master Thesis for the Ludwig Maximilian University of Munich, Alexander Friedrich Hertlein – supervised by Maximilian Högner in the group for Field-resolved Optical Precision Spectroscopy led by Ioachim Pupeza – builds on previous work by Lilienfein et al. [1], improving the experimental setup using, among others, FPGA controllers for a locking loop less sensitive to laser intensity noise and the ability for the continuous, uninterrupted and more user-friendly operation of the setup. The primary scientific goal of the thesis was to scale soliton bandwidth toward the few-cycle regime to obtain better understanding of frequency-comb-driven solitons and their potential in spectroscopy and other areas.
To achieve this, the intracavity dispersion of an enhancement cavity (EC) was measured with a new technique, before using it to establish a map of single- and multi-CS, in order to achieve deeper understanding of the main parameters in CS generation: intracavity dispersion of the EC, chirp, power, repetition rate, and offset frequency of the seeding frequency comb.
Optical frequency combs consist of electric field modes, evenly spaced in the frequency domain, typically generated by a mode-locked laser (MLL) that produces a series of ultrashort light pulses. A visual example of an optical frequency comb in the time- and frequency domains can be seen in Figure 1. The top graph shows how the carrier-envelope phase (CEP) of a laser pulse train changes by an offset between pulses, while the envelope remains constant. The bottom graph shows the “combed” frequency domain under a spectral envelope.
Figure 1: Time and frequency domain of an optical frequency comb
Enhancement cavities are a particular type of passive optical resonators, seeded with interferometric coupling, which does not actively generate light, but rather amplifies, stores, and coherently stacks it for extended periods of time. A schematic EC setup, including an input coupler (IC) and high-reflectivity mirrors (HR) in a so-called “bowtie” layout, is shown in Figure 2. Enhancement is achieved by matching the cavity length with the repetition rate of the input laser pulse train.
Figure 2: Enhancement cavity setup example
The experimental setup for this experiment first consists of the main optical elements in the form of a femtosecond laser seed frontend and a soliton generation configuration. However, for obvious reasons, we will concentrate on the central role two STEMlab125-14 units played in the comb-cavity locking section of the experiment, focusing on better CW-comb beat note detection, user-friendliness, and automated relocking. To achieve this, the original analog PID controllers were replaced by Red Pitaya FPGAs, well-known for their versatility, user-friendliness, and flexibility, with a minor hardware adjustment for improved noise characteristics. Figure 3 below shows a schematic layout of the locking scheme, including the STEMlab units. The board with the Linien software controls the CW-cavity lock, with the fast loop controlling the piezo of the CW laser, and the slow loop connected to the piezo of the cavity’s input coupler. The Red Pitaya running the DPLL software commands the MLL-CW lock, with the fast loop controlling a piezo inside the oscillator, and the slow loop regulating the crystal temperature of the CW laser.
Figure 3: Locking scheme, including 2 STEMlab125 units
The MLL-CW beat note, especially crucial for the setup, needed SNR values higher than 35 dBm at 100 kHz resolution bandwidth to ensure a stable lock. Previous setups had difficulties reaching 30 dBm, mainly due to overlapping of the MLL and the power losses generated by coupling the CW shifted copy into the same fiber. Final SNR values for the beat note showed values higher than 40 dBm. Figures 4 and 5 below show a schematic and photography of the beat note generation setup, respectively.
Figure 4: Schematic view of the beat note generation setup
Figure 5: Real image of the beat note generation setup
The results of the experiment showed that the use of the Red Pitaya FPGA controllers helped to improve the robustness of the locks and move to a new, low-phase-noise laser frontend, enabling continuous operation with automated relocking of the setup. The exclusive use of digital locks allowed a more straightforward and user-friendly locking scheme, controllable via PC.
Besides a more solid setup, further achievements, obtained during the experiment were: 1) the successful use of a cavity dispersion measurement technique to quantify the intracavity dispersion, a potentially useful tool to measure the dispersion of any highly-reflective mirror; 2) the mapping of the emerging soliton states; 3) investigation results of temporal single- and multi-soliton dynamics in build-ups, with a clear creation and destruction of single and double CS and their mutual interaction; 4) research results for nonlinear ringdowns using time-stretch dispersive Fourier transform, with the possible detection of a nonlinear characteristic in the ringdown with signatures of a red-shifted optical spectrum. Some of these results were presented at the CLEO Europe conference (26–30 June 2023 in Munich, Germany).
This scientific experiment, like most others, is open-ended, leaving space and opportunity for further improvement. New developments are already underway, with the design and manufacturing of new mirrors, as well as the introduction of new techniques to study the behavior of triple or quadruple CS interacting with each other. More options include studies to improve the noise-suppressing properties. For some good reason there seems to be no talk of replacing the STEMlab units in any further setups, and experienced Red Pitaya users are likely to know why.
[1] N. Lilienfein, C. Hofer, M. Högner, T. Saule, M. Trubetskov, V. Pervak, E. Fill, C. Riek, A. Leitenstorfer, J. Limpert, F. Krausz, and I. Pupeza, “Temporal solitons in free-space femtosecond enhancement cavities”, Nature Photonics 13, 214–218 (2019).