Red Pitaya Working in Antarctica
- Posted by
Red Pitaya Team
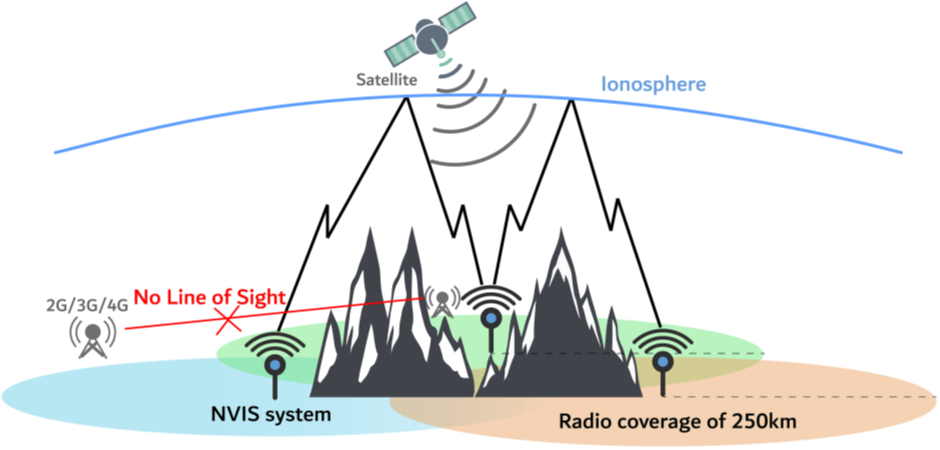
Are there any places in the world you can go without coming across a Red Pitaya board? One certainly starts to wonder, after reading the paper Sensing System for Remote Areas in Antarctica (J. Porte et al., 2020). Antarctica is about as remote as it gets, but despite this the continent has no lack of scientific bases and measuring probes. And while data transmission, along with the exponentially growing Internet-of-Things, has become something we take for granted in much of the world, the optical fiber network on the South Pole still needs some work.
Satellite communication seems the obvious solution when there are no hardlines available, although it is a rather expensive one, even without mentioning the issue of operator dependency. A cheaper and more autonomous approach is HF transmission, where radio waves are reflected by the ionosphere—the upper layers of the atmosphere, ionized by solar UV radiation. This technology allows signal transmission without line-of-sight over distances up to 3,000 km for incidence angles lower than 70°. If the geography forces you to transmit with incidence angles between 70° and 90° (Near Vertical Incidence Skywave, or NVIS), this distance drops dramatically to around 250 km, taking into account a maximum ionosphere altitude of 350 km (see the figure below).
But the technology used is not the only challenge in remote regions. The power supply is another issue, as battery-powered devices need to be used, often without the possibility of including solar panels for maximum autonomy. And as soon as you start considering batteries, you automatically think of reducing power consumption to the absolute minimum to extend runtime and minimize equipment size. Besides power consumption, a second key parameter in HF transmission is the antenna design and dimensions.
The above-mentioned paper by J. Porte et al. describes a specific experiment, relaying an HF signal on Livingston Island during the 2018-2019 Spanish Antarctic campaign, where an NVIS testbed was created between Rockie Glacier and the Spanish base camp over a distance of 5.7 km. The main goal of the experiment was to test the influence of transmission power, carrier frequency, time-of-day, bandwidth, and modulation scheme on NVIS-link performance, requiring a completely configurable SDR platform. The geographic situation, including the locations of the nodes and elevation profile, can be seen in the figures below.
The system layout, shown below, reveals a mirror design with COTS components where the setup can be used as both a transmitter and receiver, with a Red Pitaya board sitting right at the center. There, it takes care of upsampling baseband signals in transmitter mode as well as downsampling the HF carrier signal in receiver mode. The second main component is a Raspberry Pi 3 microprocessor for interaction with all peripherals.
As noted above, the second key parameter in HF transmission is the antenna design. For this experiment, an inverted V-antenna was used, including a central mast and a 2dB gain.
In transmission mode, the Raspberry sends IQ frames to the Red Pitaya board and notifications to the Teensy module (the microcontroller in charge of the control signal amplifier and antenna relay). This signal activates the Teensy transmitter mode, switching on the amplifier and commuting the antenna relay to the power amplifier. Finally, the GPS module provides time synchronization between the transmitter and receiver for sounding purposes. In receiver mode, the Red Pitaya downsamples the pickup signal from the low-noise amplifier, after which the Raspberry Pi 3 stores IQ samples on the hard drive. The system was optimized for operation at 4.3 MHz during daytime, since the ionosphere is more effective between 12:00 UTC and 19:00 UTC.
Power transmission tests showed the results reported in the figures below for second-order PSK, FSK and QAM modulations, with the Bit Error Rate (BER) vs. bit energy to noise power spectral density (EB/N0), with the received SNR calculated during postprocessing. QAM and PSK clearly show higher robustness than FSK modulation. Fourth-order modulation gave similar results, while for higher-order tests the signals received were too weak for demodulation.
Regarding the absolute results, for a transmitter power of 2 W, the probability of achieving a BER below 0.004 with fourth-order QAM was found to be 90%. When using second-order PSK with the same power, the probability increases to 94%, but with the bit rate halved. Increasing the transmission power to 24 W and using a simpler 2FSK modulation achieved similar performances.
The tests were initially performed with a bandwidth of 2.3 kHz. Higher values (up to 10 kHz) achieved lower probabilities of keeping the BER below a certain threshold, but allowed better overall performance when increasing the bit rate in a larger proportion.
This experiment has demonstrated that for low transmission power (2 W) a standard bandwidth of 2.3 kHz and 4QAM or 2PSK modulation is recommended, yielding to 4.6 or 2.3 kbps with a 90% probability of keeping the BER value below 0.004. For sensors with higher power consumption (up to 24 W), a simpler amplifier can be used with a configuration including standard bandwidth and 2FSK modulation. Higher bit rates can be achieved without increasing the power by setting the bandwidth at 5 kHz.
The results obtained in this experiment were then implemented in the real-time transmission of sensor data to the Spanish Antarctic Base with 24 W transmission power and 2.4 kHz bandwidth, thereby officially planting the Red Pitaya flag on Antarctica.