Quantum Nanomechanical Resonators, Inspired by Spiderwebs
- Posted by
Red Pitaya Team
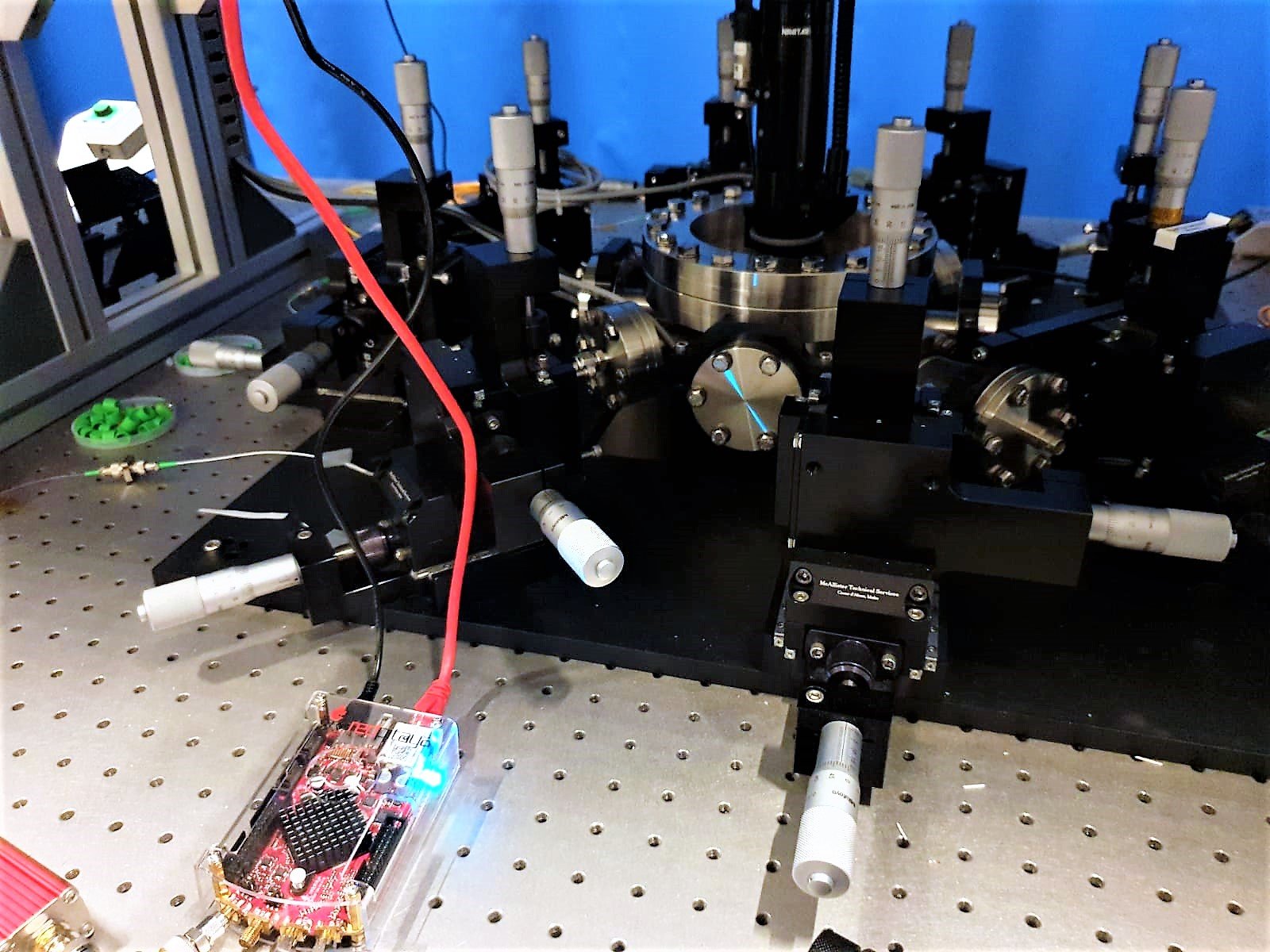
Quantum technology is clearly the way to go when you’re looking for faster, smaller and more precise high-tech solutions. Unfortunately, the extremely small size of these systems also makes them more sensitive to mechanical and other interferences from the outside world. As such, cryocooling to temperatures near absolute zero (-273.15°C) is often used to stop thermal noise from entering the systems, which leads to bulky, power-consuming devices.
But what if there was a way to mechanically isolate a system in order to avoid this interference from thermal movements? It would reduce or eliminate the need for cryogenic installations, and make the manufacturing of quantum devices much simpler and more affordable. Most previous models to do so involved 1D resonating models, mainly because of their relatively simple design rules. The degree of mechanical isolation is Qm, or the ratio of energy stored inside a resonator over the energy dissipated during one oscillation period. In terms of quantum technology, this is the average number of coherent oscillations a nanomechanical resonator can undergo before one phonon (quanta of vibration) of thermal noise enters and generates decoherence of the resonator’s quantum properties. In order to optimize the Qm ratio, a team of researchers at TU Delft looked for inspiration in one of nature’s mechanical marvels: the spiderweb. Experts agree that, on top of their impressive structural properties with regard to toughness and stiffness, they are remarkable vibration sensors. A web allows a spider to detect movement inside the web, but not outside it, making it an outstanding mechanically isolated environment.
With this in mind, different simulation configurations of web-shaped structures were run using Bayesian optimization, considering beams or threads, made of highly stressed Si3N4, which can be as thin as 20 nm (compared to the mm-range thickness of spiderweb threads). This resulted in an optimum quality factor Qm greater than 1.75 billion at 134.9 kHz, achieved by the deceptively simple structure that can be seen in the figure below.
Also surprisingly, this quality factor is reached without the need for tether widths smaller than a micrometer, which allows faster and cheaper manufacturing. The nanomechanical resonators were fabricated from 58 nm-thick high-stress (1.07 GPa) Si3N4, deposited on a silicon substrate by means of LPCVD (Low Pressure Chemical Vapor Deposition).
In order to verify the performance and compare it with the simulation results, a test setup was designed to experimentally determine the system’s quality factor from a ringdown measurement, with the resonator’s amplitude decay rate as an indicator of energy dissipation and the mechanical quality factor. All this was done under a vacuum (P < 10-8 hPa) to avoid air damping. The schematic representation of the fabrication process flow and the mechanical characterization setup can be seen below.
All measurements were performed using a custom balanced homodyne detection interferometer. The mechanical displacement was probed with a fiber-coupled infrared laser (1550 nm). A piezoelectric plate was connected to the sample holder to actuate the devices mechanically. The phase of the local oscillator signal was controlled with a fiber stretcher, driven by a PID-controller. This function was performed by Red Pitaya’s versatile FPGA-board, the STEMlab 125-14, with its flexible I/O-configuration. The interferometer’s low frequency fluctuations were stabilized, using the signal measured from the balanced photodetector, as an error signal for a feedback loop. Thermomechanical noise spectra were acquired with an electronic spectrum analyzer, without mechanical excitation applied to the piezoelectric plate.
The experiment has shown that ultralow dissipation in room-temperature environments can be achieved via a simulation-based optimization approach for the design of a spiderweb-shaped nanomechanical resonator, enabling high-Qm devices with smaller aspect ratios compared to previous designs, which makes it easier, cheaper and faster to manufacture them. This can only strengthen the belief that affordable quantum technology is waiting just around the corner, and obviously, the ever more affordable and reliable Red Pitaya units will be there to help out wherever possible.
Sources:
TU Delft creates one of the world’s most precise microchip sensors – thanks to a spiderweb